Images of a bacterial motor show how it can reverse direction with the flip of a biological switch.
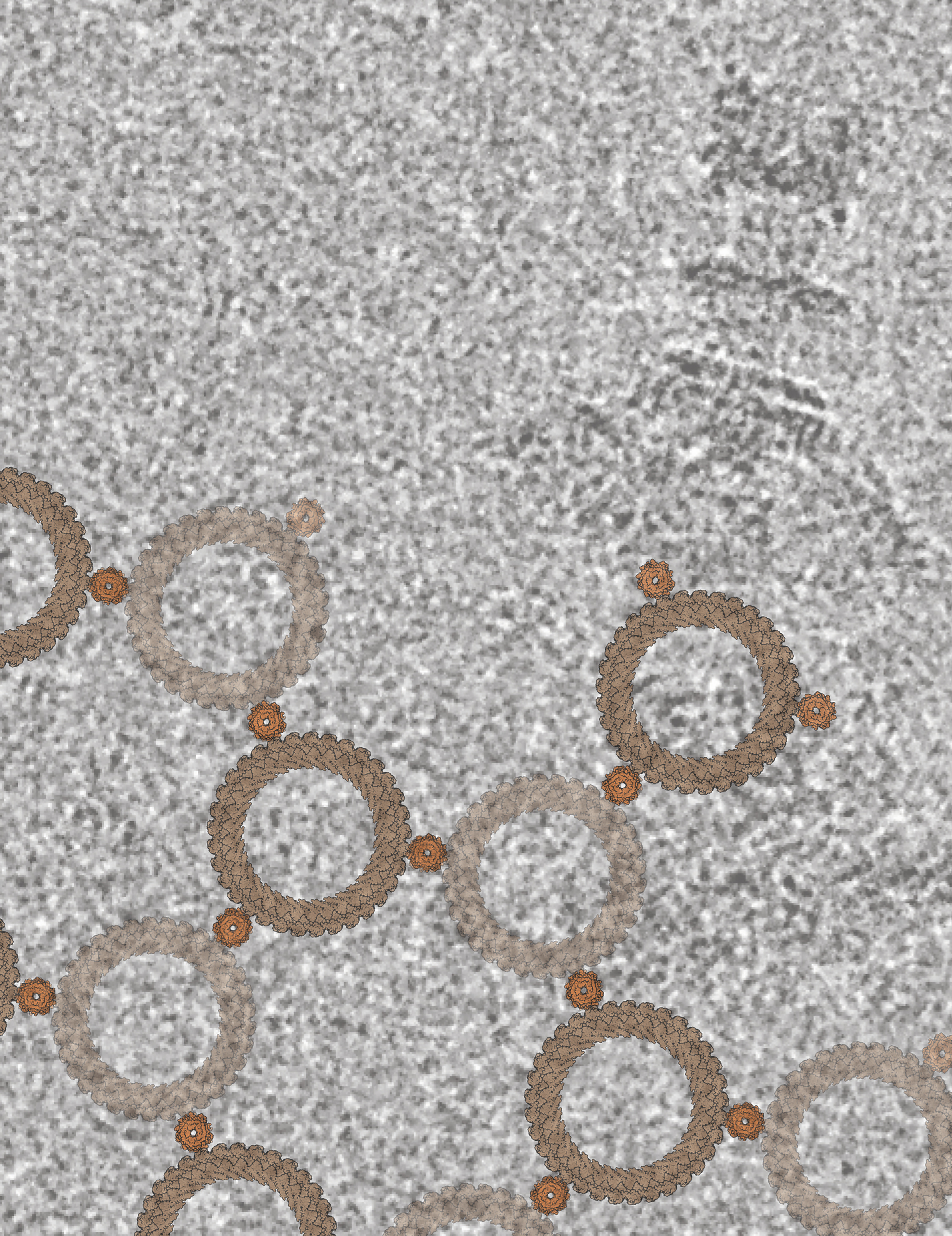
The background shows a field of view from a cryo-electron microscopy-generated micrograph with a single Salmonella flagellar basal body — the flagellar motor — visible in the top right (darker gray). Overlaid on the image is a lattice created from atomic models of the C-ring (larger ring in tan) and the stator (smaller ring in orange), which are responsible for driving the rotation of bacterial flagella.
Credit: Steven Johnson, CCR, NCI, NIH
Many bacteria, including pathogenic ones, propel themselves with whip-like appendages called flagella. Rotations of the flagella keep a bacterium moving steadily forward — sometimes toward food, sometimes away from toxins. With the flip of a biological switch, the rotary motor that powers a flagellum can be reversed, setting the microbe off in a new direction — potentially toward a more hospitable environment.
Striking new images of the motor that powers this movement, captured in the lab of Senior Investigator Susan Lea, D.Phil., and published in Nature Microbiology, show how the motor reconfigures itself to change direction. “The changes that we see between the states which go in one direction and the states which go in the other direction are huge, huge conformational changes — much bigger than we might have predicted would be possible without tearing an object apart,” Lea says. That physical transformation enables the motor to turn both clockwise and counterclockwise.
Scientists have struggled to explain this directional shift, because flagella are powered by the flow of ions across the cell membrane, which typically move in only one direction. And it is hard to get a close look at the system to learn how it works. The flagellar motor, which is made up of dozens of proteins, is fragile, dynamic and, as biological complexes go, massive in size. These features make it difficult for structural biologists to visualize the complex at a molecular level.
Steven Johnson, D.Phil., and Justin Deme, Ph.D., Staff Scientists in Lea’s lab, solved the problem using a high-resolution imaging method called cryo-electron microscopy. They captured about 85,000 images of the bacterial motor, viewing it from every angle and in both of its forms: positioned to move clockwise or counterclockwise. Lea points out that her team needed the vast computing power of the Frederick Research Computing Environment, made available through NCI, to assemble the data into complete three-dimensional structures.
In their final models, the ion-powered motor and a smaller adaptor that links the motor to the flagellum appear as a pair of cogs that fit together in two dramatically different ways. In one configuration, the smaller cog rotates along the outer edge of the larger cog. In the other, a change in the shape of one of the proteins in the complex moves the small cog inside the larger cog. This rearrangement means that while the motor that drives the movement always rotates in the same direction, the flagellum-turning cog can move in either direction, depending on where it sits.
“If you compared the two states, that little piece literally flipped 180 degrees over. That's a rare thing for a protein to do,” Lea says. “It’s a pleasingly simple answer, but it was incredibly complex to get there.”
Lea says her team’s discovery not only shows that proteins are capable of extreme conformational change, it also exposes a fundamental aspect of microbial biology that might one day inform the design of new kinds of antibiotics. Because the core components of bacterial flagella are very similar across species, what they have learned is expected to apply to many types of bacteria. “A lot of cancer patients die of bacterial infections, so we think it is really important for cancer,” she says.