The architectural details of a notorious cancer-promoting protein offer clues about how to keep it safely switched off.
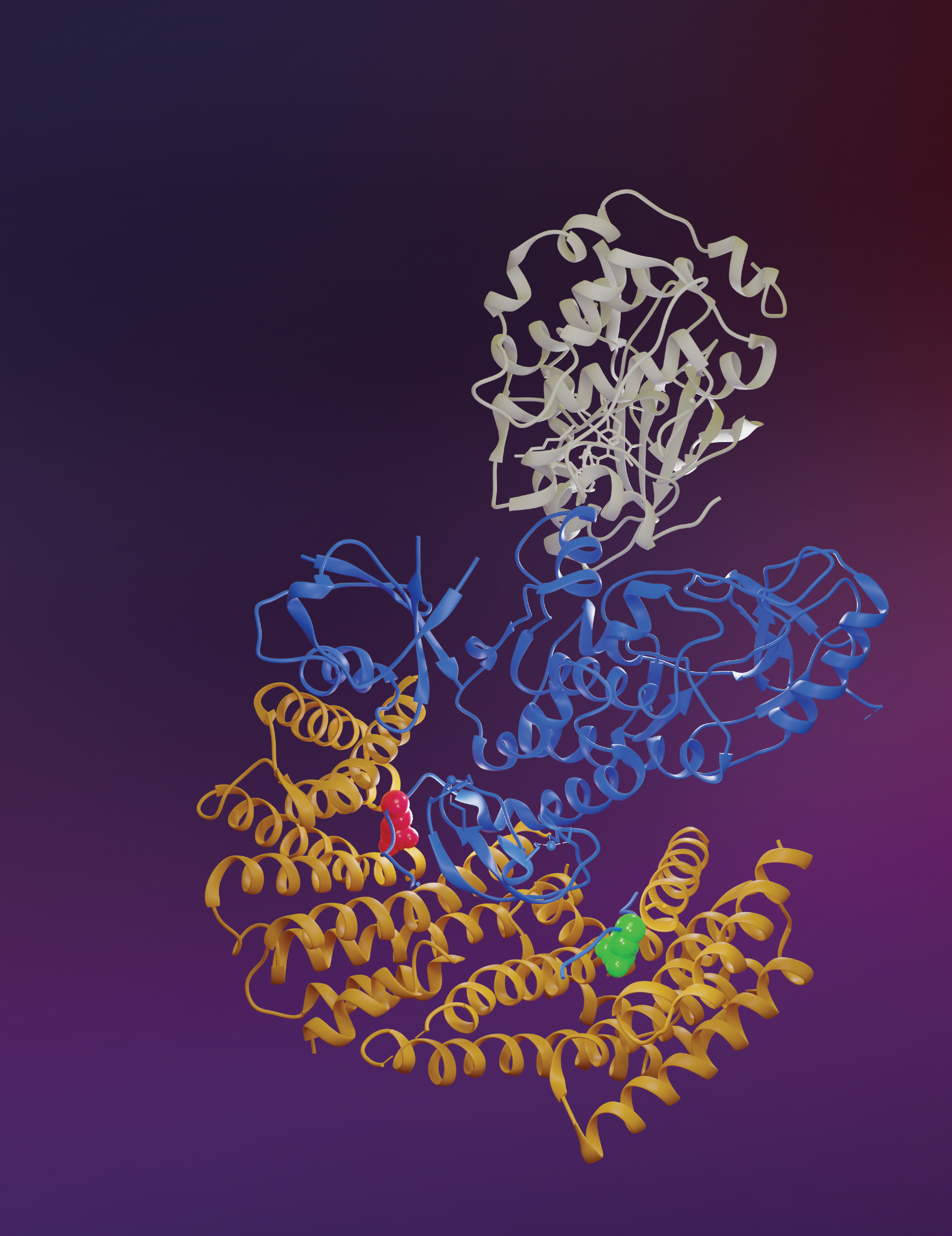
BRAF and other RAS pathway proteins are notorious in cancer development. This 3D structure shows inactive BRAF (blue, middle) bound to MEK (gray, top) and two 14-3-3 proteins (yellow, bottom). The 14-3-3 proteins bind to the pS729 (red) and pS365 (green) sites in the BRAF protein. Knowing how these proteins bind together in such high resolution may help find ways to stop the BRAF protein from triggering cancerous cell growth. Credit: Juliana A. Martinez Fiesco, David E. Durrant, Deborah K. Morrison and Ping Zhang, CCR, NCI, NIH; Joseph Meyer, SPGM, FNL, NCI, NIH
The growth of many skin cancers is driven by a mutated, overactive version of a protein called BRAF. Even without mutations in the gene, BRAF helps drive excessive growth of cancer cells, because it is an essential partner of another commonly mutated, growth-promoting protein called RAS. The RAS protein is one of the most common drivers of human cancer — but it can only stimulate cell growth with the help of BRAF.
Senior Investigator Deborah Morrison, Ph.D., and Stadtman Investigator Ping Zhang, Ph.D., have now used sophisticated atomic imaging methods to reveal the structure of BRAF in two distinct states: in its active and inactive forms. Those studies, reported in Nature Communications, are the first to depict the full BRAF protein at atomic resolution. The data show how the protein dramatically reconfigures itself as it shifts from its inactive to active form — an insight that could help guide the design of a drug that could lock BRAF into its inactive state so that it can no longer drive the growth of cancer cells.
Morrison’s team, which has studied BRAF for decades, was able to produce BRAF in animal cells and extract it in association with members of the 14-3-3 protein family. Then Zhang and her team used cryo-electron microscopy to take a series of very high-resolution snapshots, capturing the molecules from all angles.
BRAF molecules work in pairs. When cell growth is not needed, they remain separate, folded up into their inactive form. 14-3-3 proteins act as a molecular clamp that locks each BRAF molecule into this inhibited state. Interaction with RAS disrupts that configuration, freeing the BRAF molecules to pair up and relay growth signals through the cell, and in this way contributes to cancerous cell growth.
Deborah K. Morrison, Ph.D.
Chief
Laboratory of Cell and Developmental Signaling
Ping Zhang, Ph.D.
NIH Stadtman Investigator
Center for Structural Biology
With the new electron microscopy images, Zhang and Morrison’s teams were able to assemble three-dimensional structures of BRAF in both its solitary, inhibited state and in its active, paired-up form. Those structures reveal some unexpected details about how autoinhibited BRAF transitions into its active state when it interacts with RAS. For example, it appears that RAS may shove the 14-3-3 protein aside, enabling BRAF to unfold into a shape that can grab onto another BRAF molecule.
“We have a picture of the starting and end points,” says Zhang. “From here we can understand how RAS binds to the autoinhibited BRAF as a whole complex and how that binding can initiate BRAF activation by releasing it from its autoinhibitory state.”
Zhang and Morrison are excited about these findings because their new structures could help drug developers figure out how to block the interaction between BRAF and RAS. Knowing the precise configurations of these key cancer players could also suggest ways to halt BRAF’s activity so it can no longer trigger cell growth, with or without RAS. “If you could find a way to stabilize the autoinhibited state, that could be useful in drug design,” Morrison says.