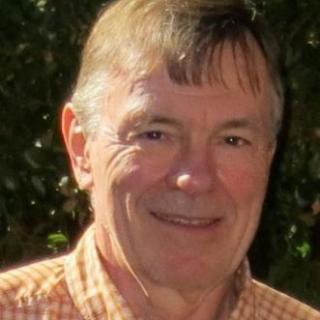
Donald L. Court, Ph.D.
- Center for Cancer Research
- National Cancer Institute
- Building 558, Room 4
- Frederick, MD 21702-1201
- 301-846-5940
- courtd@mail.nih.gov
RESEARCH SUMMARY
Dr. Court developed recombineering as a revolutionary way to engineer DNA in living cells and has defined its mechanism; discovered several gene regulatory mechanisms that describe the systems biology of phage λ and Escherichia coli (E. coli); defined structures and action mechanisms for the DICER homolog RNaseIII, and identified the Era-GTPase as a cell-cycle check-point regulator in E. coli that is present from bacteria to mammals.
Areas of Expertise
Research
Developmental systems are controlled by modulating gene expression in response to internally programmed signals as well as to external signals. Our laboratory was interested in studying the molecular interactions and the signaling that occur to regulate gene expression. We exploited the genetic systems available in Escherichia coli (E. coli), its plasmids, and its viruses (e.g., bacteriophage lambda) to help us understand (1) gene regulation at the levels of transcription initiation and elongation, and translation initiation, and (2) cell growth and cell cycle control signals.
RECOMBINEERING: We developed an in vivo cloning and gene modification system using lambda Red-mediated homologous recombination with short (<50 bp) homologies. This technology, called recombineering, was used as a great tool in E. coli for genetic modification. Over the years, we provided recombineering strains to more than 3,000 labs. Well over half of these labs worked on eukaryotic model systems like mouse and Drosophila. Modifications were made to clones in E. coli, which were then used to generate transgenics or targeted gene modifications in these model systems.
Recombineering eliminated the need to cut DNA with restriction enzymes or join DNA fragments with DNA ligase. This extremely efficient recombination system revolutionized the way in which recombinant DNA work was done and became a major tool in the field of synthetic biology. Visit our website on recombineering: https://redrecombineering.ncifcrf.gov/
The N gene of lambda is the first gene expressed following viral infection. The function of the N protein is necessary for expression of most other lambda genes by its actions as a positive regulator. Positive activation of other genes occurs by N binding to specific RNA sites called NUT, modifying the RNA polymerase transcription complex. This modified polymerase complex reads through transcription terminators to distal lambda genes. Thus, the expression and action of N are central to the control of lambda development.
We determined that N is subject to novel posttranscriptional regulatory circuits. Expression of the N gene is autoregulated by N binding to the NUT RNA site 150 bases upstream of the N gene, thereby repressing the translation of N more than 100-fold over this long distance. The N-modified RNA polymerase complex is required for translational repression. Thus, antitermination and translation repression by N are coupled. This may be caused by a specific folding of the RNA structure that brings the NUT RNA into close juxtaposition with the N ribosome-binding site. RNaseIII, an endonuclease, recognizes the stem structure and cleaves it, separating NUT from the N RNA. This cleavage prevents N translational repression but actually enhances antitermination, presumably by releasing the antitermination complex from its interaction with the NUT RNA.
Additionally, we found that RNaseIII is expressed from an operon in which an essential low-molecular-weight GTP-binding protein, Era, is also encoded. From this operon, RNaseIII and Era expression is coordinately regulated and increases in relation to growth rate. This growth rate regulation of RNaseIII and Era occurs at the posttranscriptional level, but the mechanism remains unknown. The accumulation of adequate levels of Era is essential for cytokinesis to be completed and cell growth to continue. We speculated that a threshold level of Era must accumulate before Era-GTPase is activated by a cellular signal to cause cell division and to allow cell growth to continue. We believed RNaseIII and Era were key components that couple regulation of growth and the cell cycle.
Our collaborators included David Friedman, University of Michigan; Max Gottesman, Columbia University; William Margolin, University of Texas-Houston Medical School; Joel Stavans, Weizmann Institute of Science; and Sankar Adhya, Stuart Austin, Xinhua Ji, Ding Jin, Mikhail Kashlev, and Jeffrey Strathern, CCR.
Publications
- Bibliography Link
- View Dr. Court's PubMed Summary.
Examining a DNA Replication Requirement for Bacteriophage λ Red- and Rac Prophage RecET-Promoted Recombination in Escherichia coli
tCRISPRi: tunable and reversible, one-step control of gene expression
Location of the unique integration site on an Escherichia coli chromosome by bacteriophage lambda DNA in vivo
Bacteriophage λ N protein inhibits transcription slippage by Escherichia coli RNA polymerase
Bacterial DNA polymerases participate in oligonucleotide recombination
Biography
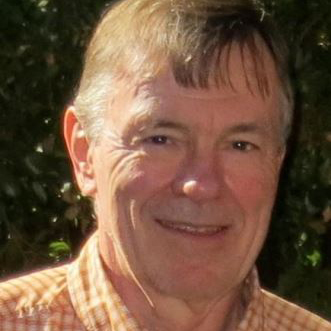
Donald L. Court, Ph.D.
Dr. Court received his Ph.D. from the University of Rochester, Department of Biology. He was a research associate at Stanford University and the University of California-Berkeley in the laboratories of Drs. Alan Campbell and Harrison Echols, respectively, before joining the NIH and the National Cancer Institute in 1972. In 1988, Dr. Court joined the Frederick ABL-Basic Research Program as Chief of the Molecular Control and Genetics Section, and in 1999, he joined the Center for Cancer Research of the National Cancer Institute.
Dr. Court retired as a Senior Investigator in 2020 and is now an NIH Scientist Emeritus.
Covers
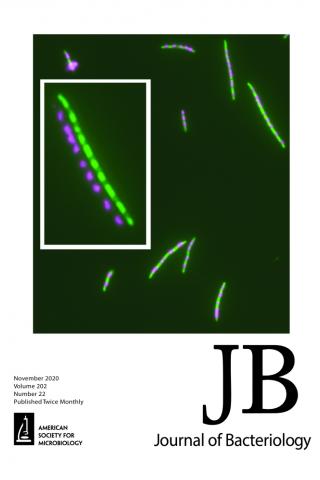
Overproduction of a Dominant Mutant of the Conserved Era GTPase Inhibits Cell Division in Escherichia coli
Cell growth and division are coordinated, ensuring homeostasis under any given growth condition, with division occurring as cell mass doubles. The signals and controlling circuit(s) between growth and division are not well understood; however, it is known in Escherichia coli that the essential GTPase Era, which is growth rate regulated, coordinates the two functions and may be a checkpoint regulator of both. We have isolated a mutant of Era that separates its effect on growth and division. When overproduced, the mutant protein Era647 is dominant to wild-type Era and blocks division, causing cells to filament. Multicopy suppressors that prevent the filamentation phenotype of Era647 either increase the expression of FtsZ or decrease the expression of the Era647 protein. Excess Era647 induces complete delocalization of Z rings, providing an explanation for why Era647 induces filamentation, but this effect is probably not due to direct interaction between Era647 and FtsZ. The hypermorphic ftsZ* allele at the native locus can suppress the effects of Era647 overproduction, indicating that extra FtsZ is not required for the suppression, but another hypermorphic allele that accelerates cell division through periplasmic signaling, ftsL*, cannot. Together, these results suggest that Era647 blocks cell division by destabilizing the Z ring.
Xiaomei Zhou, Howard K. Peters III, Xintian Li, Nina Costantino, Vandana Kumari, Genbin Shi, Chao Tu, Todd A. Cameron, Daniel P. Haeusser, Daniel E. Vega, Xinhua Ji, William Margolin, Donald L. Court See related article in Journal of Bacteriology November 2020, vol. 202, no. 21, e00342-20.
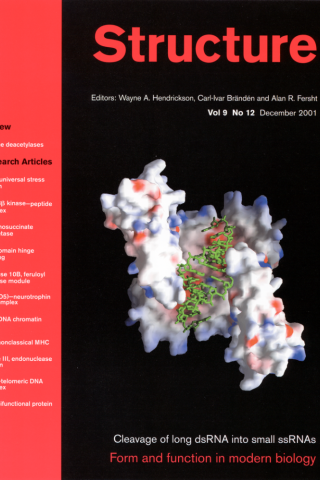
Crystallographic and Modeling Studies of RNase III Suggest a Mechanism for Double-Stranded RNA Cleavage
Background: Ribonuclease III belongs to the family of Mg2+-dependent endonucleases that show specificity for double-stranded RNA (dsRNA). RNase III is conserved in all known bacteria and eukaryotes and has 1–2 copies of a 9-residue consensus sequence, known as the RNase III signature motif. The bacterial RNase III proteins are the simplest, consisting of two domains: an N-terminal endonuclease domain, followed by a double-stranded RNA binding domain (dsRBD). The three-dimensional structure of the dsRBD in Escherichia coli RNase III has been elucidated; no structural information is available for the endonuclease domain of any RNase III.
Results: We present the crystal structures of the RNase III endonuclease domain in its ligand-free form and in complex with Mn2+. The structures reveal a novel protein fold and suggest a mechanism for dsRNA cleavage. On the basis of structural, genetic, and biological data, we have constructed a hypothetical model of RNase III in complex with dsRNA and Mg2+ ion, which provides the first glimpse of RNase III in action.
Conclusions: The functional RNase III dimer is formed via mainly hydrophobic interactions, including a “ball-and-socket” junction that ensures accurate alignment of the two monomers. The fold of the polypeptide chain and its dimerization create a valley with two compound active centers at each end of the valley. The valley can accommodate a dsRNA substrate. Mn2+ binding has significant impact on crystal packing, intermolecular interactions, thermal stability, and the formation of two RNA-cutting sites within each compound active center.
Blaszczyk J, Tropea JE, Bubunenko M, Routzahn KM, Waugh DS, Court DL, Ji X. Structure. 2001 Dec;9(12):1225-36. PMID:1173804